my services
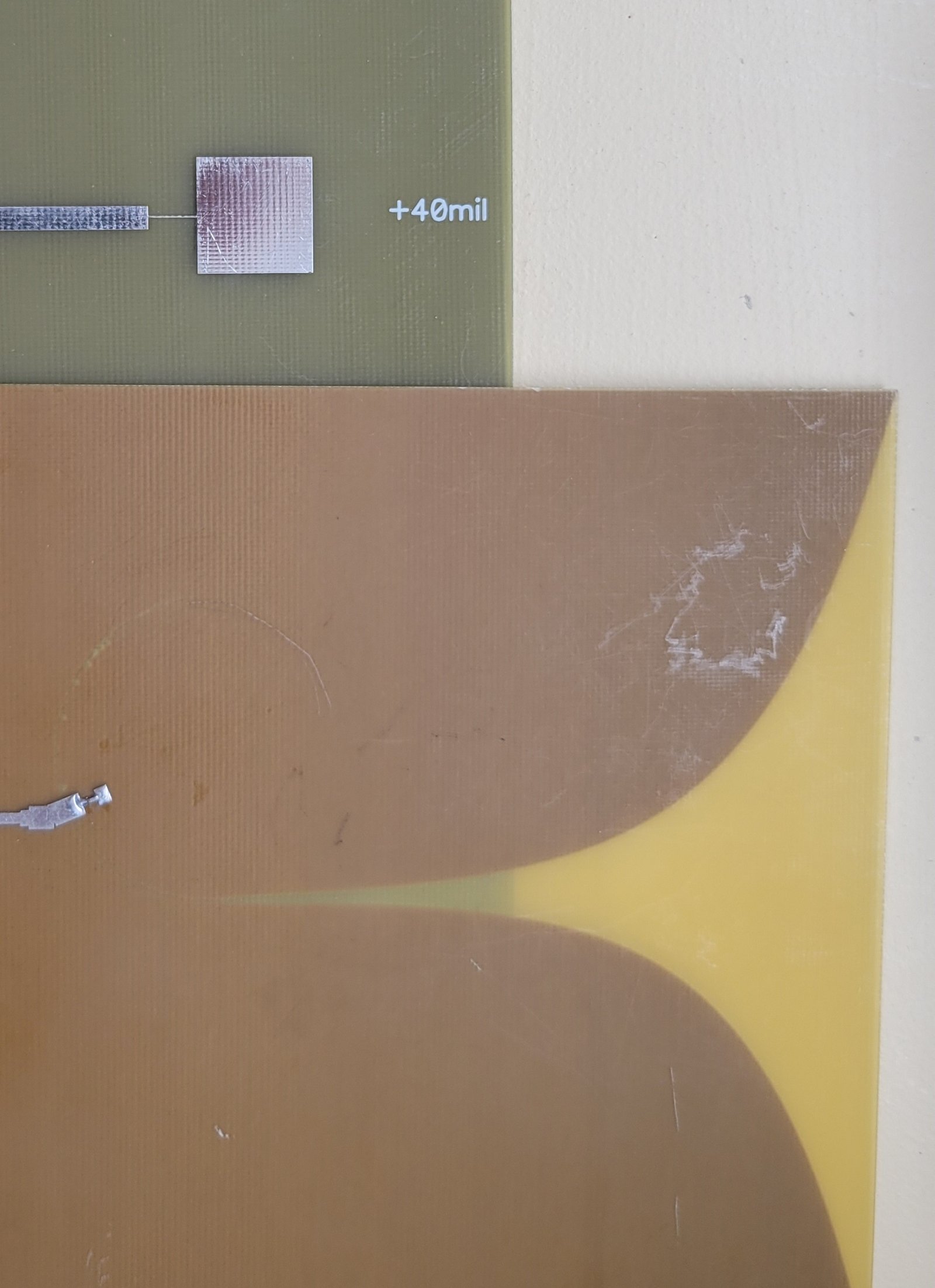
Antenna Design Service
You have a need for a high performance antenna that meets your size, cost, and performance needs. But you don’t have the time or the engineering talent required to develop it. I understand and have tailored a process specifically for your needs.
The process provides expert engineering services in designing high-performance antenna elements customized to meet your unique needs. Whether it’s meeting gain requirements, optimizing bandwidth, or achieving precise performance specifications, my designs are crafted with a focus on quality, reliability, and innovation.
Each solution is tailored to empower your systems, ensuring they operate at peak efficiency and deliver exceptional results. With years of experience and a passion for excellence, I work closely with clients to address challenges, accelerate development cycles, and exceed expectations. Let my antenna design expertise transform your project into a cutting-edge solution that performs flawlessly in any application.
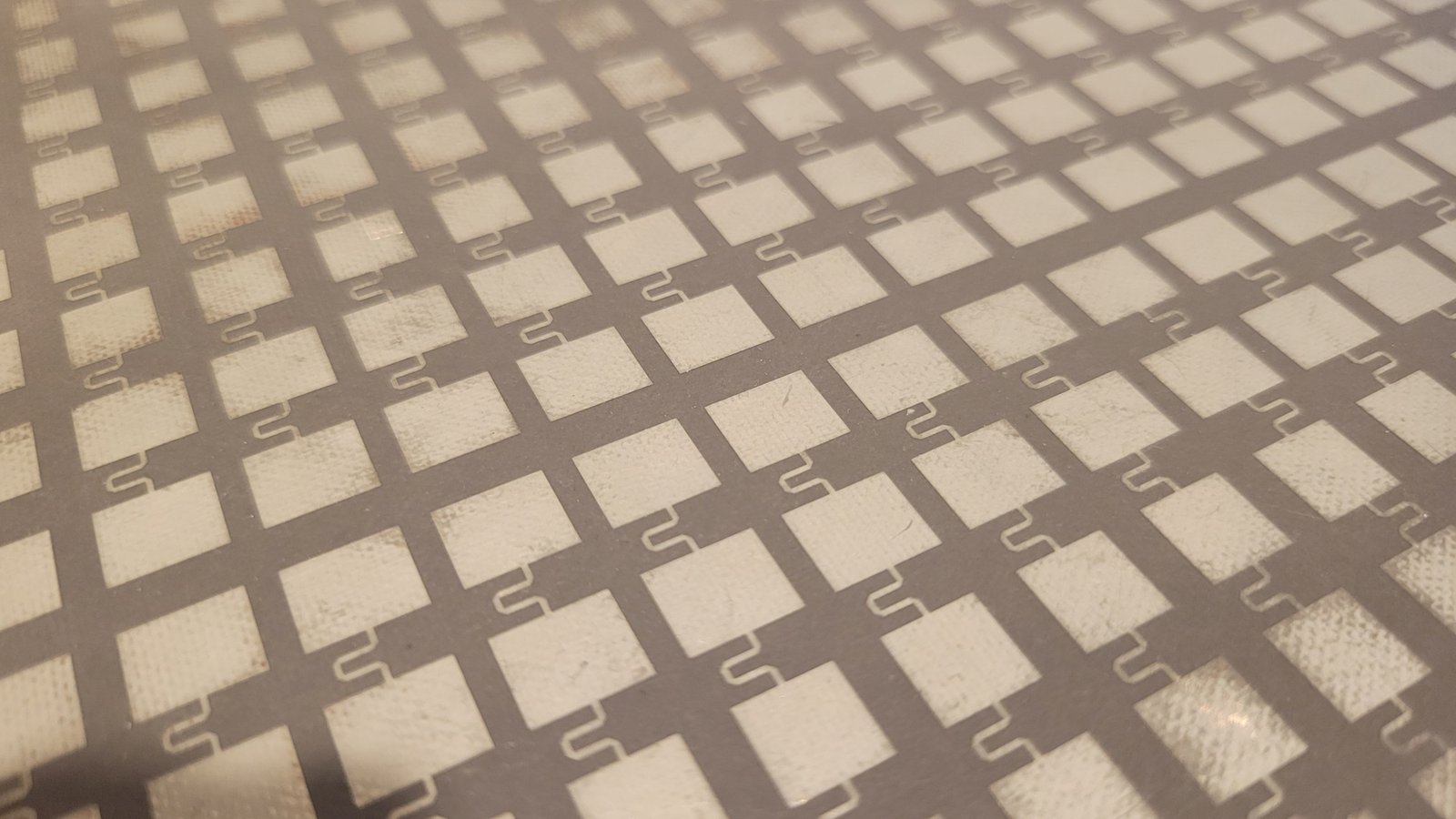
Phased Array Design Service
Your phased array requirements may seem unclear or challenging for you too meet in the timeline required. My proven phased array development process will help guide you to the solution.
From concept to deployment, the process is crafted to result in innovative designs that optimize beamforming, coverage, and signal performance.
The phased array solutions are tailored to deliver precision, reliability, and efficiency, ensuring your systems excel in complex environments. With a focus on streamlined development cycles and cutting-edge technology, I address your specific challenges while delivering superior results.
Whether for defense, aerospace, or telecommunications, my expertise ensures your phased array systems achieve unparalleled performance and a competitive edge. Let’s bring your vision to life.
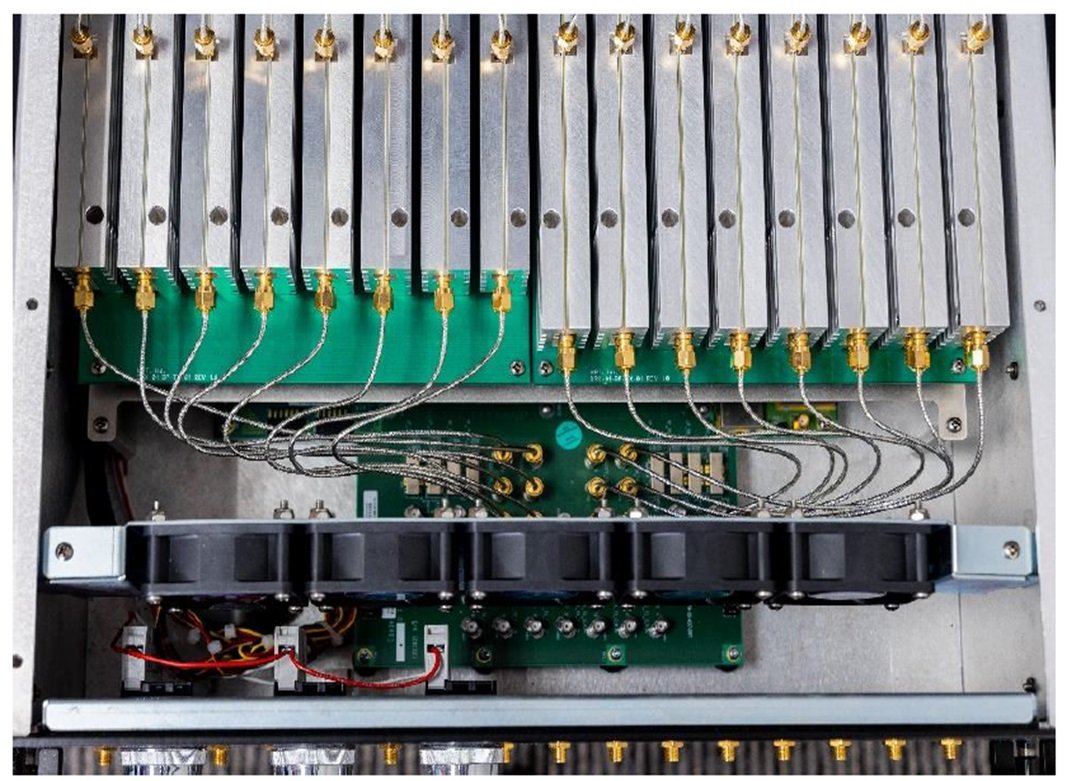
System Design Service
Complex RF system solutions require the experience and knowledge to perform trade studies, system architecture, knowledge of proven solution performance, and the ability to design specification compliant solutions.
My comprehensive engineering design services for RF systems, spanning architecture, design, prototyping, and transition to production.
My expertise includes conceptualizing and developing robust RF architectures tailored to meet specific project requirements. I excel in designing and testing prototypes, ensuring seamless integration of components and optimizing system performance.
With a deep understanding of RF principles and industry standards, I guide projects from initial concept through to production, managing risks and maintaining quality.
I collaborate closely with cross-functional teams, ensuring smooth transitions from prototype to production, while adhering to timelines, budgets, and regulatory standards for reliable, scalable RF solutions.
Antenna Testing And Characterization
Using a combination of internal testing capability and trusted third party test labs, I am able to fully characterize antenna elements, phased arrays, and other antenna systems. Below is a partial list of the measurement services that are offered.
- Antenna Radiation Patterns
- Gain of Antennas and Phased Arrays
- Radiation Efficiency and Aperture Efficiency
- Beam Width
- Impedance Match/Return Loss
- Side Lobe Levels ... and more
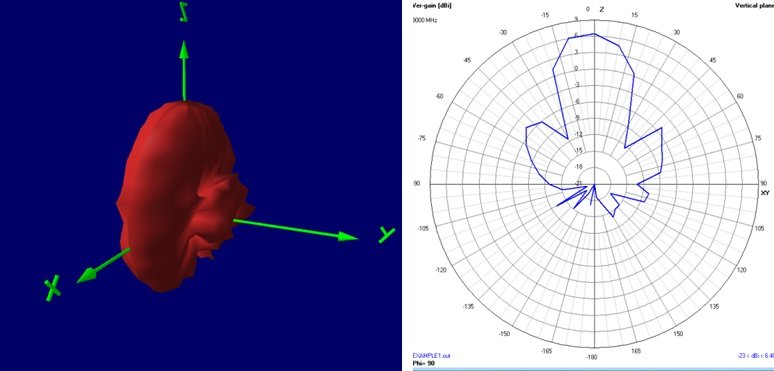
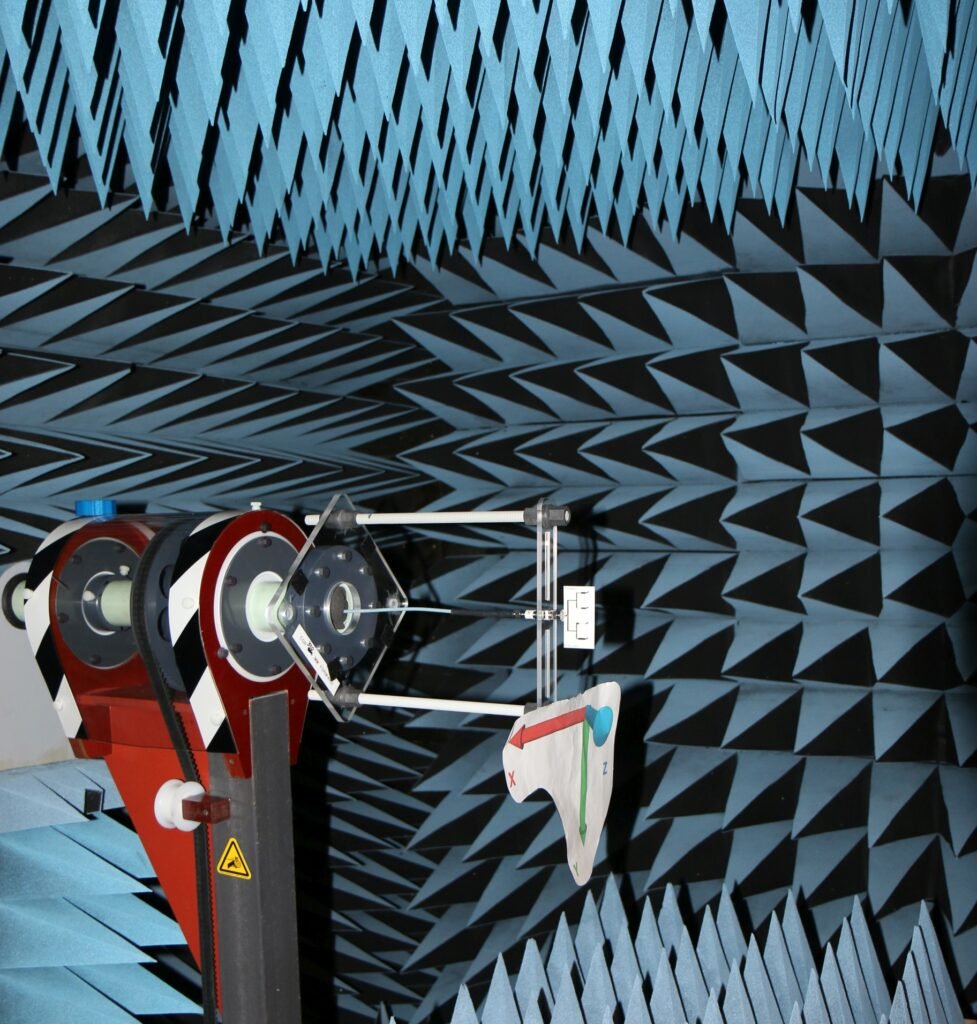
Image of one of my antenna designs being measured for radiation pattern, gain, efficiency, and other parameters in an anechoic chamber.
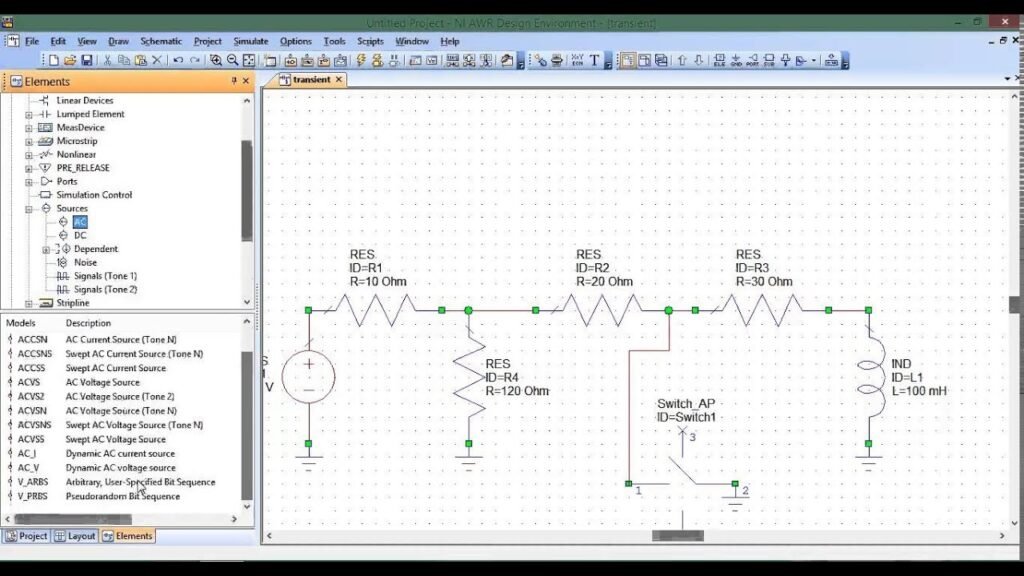
Example Microwave Circuit Simulation
I Use The Most Advanced Antenna Design EDA Tools
- Linear and Non-Linear Microwave Circuits
- EM Simulation Using FEM and FDTD Techniques
- Spice Simulation
- Amplifiers, Filters, Couplers, Switches, and more ...
- Bias, Control, and Analog Circuits ... and more